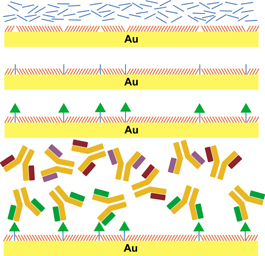
Capturing Large-Molecule Fish with Small-Molecule
Bait.
A single layer of molecules (red lines) that
resists biomolecule binding is first self-organized on a gold
substrate. This film has inherent defects and when it is placed in
a solution of tether molecules (blue lines), they insert
themselves into the holes. Next, bait molecules (green triangles)
are linked to the tethers. When the surface is exposed to
different proteins, only those with high affinity for the bait
(color-coded green Y�s) bind to the surface. Spacing the molecular
fishing rods at appropriate distances overcomes problems
associated with selective recognition between large biomolecules
and small ligands. In the example described, the small bait is
serotonin, a neurotransmitter important in depression and anxiety,
and the large fish are antibodies. In the future, this type of
surface will be used to capture brain receptor proteins or
synthetic sensors.
Credit: Penn State
|
The process starts with a self-assembled monolayer (SAM), a
single-molecule-thick layer that organizes itself on a surface. The
molecules that make up the SAM terminate in and expose
oligoethyleneglycol units that are known to prevent adhesion of
proteins and other large biomolecules. Next, tether molecules are
inserted into the defects that naturally occur in the SAM. Finally, a
small molecule, in this case the neurotransmitter serotonin, is
chemically linked to the tether molecules. Since the defects in the
SAM occur at irregular but controllable intervals, serotonin molecules
are prevented from clumping together. This is key to their being
recognized by the correct proteins.
When the surface is exposed to a solution containing many different
proteins, only those with high affinities for the tethered small
molecule selectively attach to the surface. The bound protein
molecules can then be identified in place or removed for
characterization. "The tethered neurotransmitter acts like a fishing
pole," says Andrews. "When the small molecule 'bait' is correctly
placed on the surface, it captures much larger molecules that interact
with it in a biologically specific way."
As a result of this inherent selectivity, it is possible to identify
biomolecules, by function, from a sea of thousands of different types
of molecules. Weiss adds, "The key to obtaining a highly specific
association is producing optimal spacing of the tethered
neurotransmitters. The ideal spacing allows large molecules to
recognize the functional groups of the small molecule while avoiding
nonspecific binding to the surface itself."
Because of their selectivity, these materials are suitable for a
variety of investigations in biological systems. "Each
neurotransmitter can bind to a number of different receptors in the
brain," says Andrews. "Some of these receptors are known, but there
are many more to identify. Also, the numbers of receptors are altered
in different disease states and in response to treatment, and these
capture surfaces could be used to study how groups of functionally
related proteins change in a coordinated fashion."
The research team includes Mitchell Shuster, graduate student in
physics, Amit Vaish, graduate student in bioengineering, Matthew
Szapacs, then a graduate student in chemistry and now a research
scientist at GlaxoSmithKline, and Beth Anderson, then a graduate
student in chemistry and now a postdoctoral fellow. The work is a part
of and supported by the Center for Nanoscale Science at Penn State, a
National Science Foundation Materials Research Science and Engineering
Center.
|