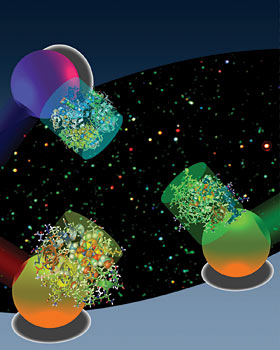
Lights scatter from metallic nanoplasmonic
particles upon excitation of an external light source. UC Berkeley
researchers coupled the metallic nanoparticles with biomolecules
to detect chemical signals within a single living cell at
unprecedented resolution.
(Graphic by Gang Logan Liu and Luke Lee/UC
Berkeley)
|
To study the biochemical processes of a cell, scientists currently cut
through its outer membrane to separate and analyze the cellular
components. That method can never provide a real-time view of how
components function together because the cell is killed in the process
of extracting its components.
"Until now, there has been no non-invasive method that exists that can
capture the chemical fingerprints of molecules with nanoscale spatial
resolution within a single living cell," said Lee, who is also a
faculty affiliate of the California Institute for Quantitative
Biosciences and the co-director of the Berkeley Sensor and Actuator
Center. "There is great hope that stem cells can one day be used to
treat diseases, but one of the biggest challenges in this field is
understanding exactly how individual cells differentiate. What is
happening inside a stem cell as it develops into a heart muscle
instead of a tooth or a strand of hair? To find out, we need to look
at the telltale chemical signals involved as proteins and genes
function together within a cell."
The researchers tackled this challenge by improving upon conventional
optical absorption spectroscopy, a technique by which light is passed
through a solution of molecules to determine which wavelengths are
absorbed. Cytochrome c, for instance, is a protein involved in cell
metabolism and cell death that has several optical absorption peaks of
around 550 nanometers.
The absorption spectra of a molecule can change based upon the
chemical changes that occur as it interacts with other molecules, such
as oxygen.
"For conventional optical absorption spectroscopy to work, a
relatively high concentration of biomolecules and a large volume of
solution is needed in order to detect these subtle changes in
frequencies and absorption peaks," said Lee. "That's because optical
absorption signals from a single biomolecule are very weak, so you
need to kill hundreds to millions of cells to fish out enough of the
target molecule for detection."
The researchers came up with a novel solution to this problem by
coupling biomolecules, the protein cytochrome c in this study, with
tiny particles of gold measuring 20-30 nanometers long. The electrons
on the surface of metal particles such as gold and silver are known to
oscillate at specific frequencies in response to light, a phenomenon
known as plasmon resonance. The resonant frequencies of the gold
nanoparticles are much easier to detect than the weak optical signals
of cytochrome c, giving the researchers an easier target.
Gold nanoparticles were chosen because they have a plasmon resonance
wavelength ranging from 530 to 580 nanometers, corresponding to the
absorption peak of cytochrome c.
"When the absorption peak of the biomolecule overlaps with the plasmon
resonance frequency of the gold particle, you can see whether they are
exchanging energy," said study co-lead author Gang Logan Liu, who
conducted the research as a UC Berkeley Ph.D. student in
bioengineering. "This energy transfer shows up as small dips,
something we call 'quenching,' in the characteristic absorption peak
of the gold particle."
A relatively small concentration of the molecule is needed to create
these quenching dips, so instead of a concentration of millions of
molecules, researchers can get by with hundreds or even dozens of
molecules. The sensitivity and selectivity of the quenching dips will
improve the molecular diagnosis of diseases and be instrumental in the
development of personalized medicine, the researchers said.
The researchers repeated the experiment matching the protein
hemoglobin with silver nanoparticles and achieved similar results.
"Our technique kills two birds with one stone," Lee said. "We're
reducing the spatial resolution required to detect the molecule at the
same time we're able to obtain chemical information about molecules
while they are in a living cell. In a way, these gold particles are
like 'nano-stars' because they illuminate the inner life of a cellular
galaxy."
Other researchers on the UC Berkeley team are Yi-Tao Long, co-lead
author and postdoctoral scholar in bioengineering; Yeonho Choi, a Ph.D.
student in mechanical engineering; and Taewook Kang, a postdoctoral
scholar in bioengineering.
The Ministry of Science and Technology in Korea helped support this
research.
|