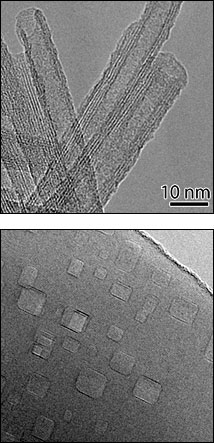
Transmission electron micrographs
of nanocavity-filled titanate nanorods (bottom) and iron-doped
titanium oxide nanotubes (top). Both are being investigated as
photocatalysts for reactions to produce hydrogen gas. The improved
light-absorption of the nanocavity-filled nanorods also makes them
ideal new materials for sunscreen.
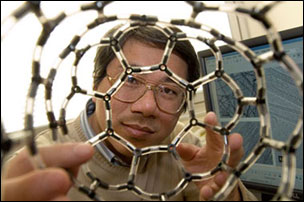
Wei-Qiang Han |
"Titanium dioxide's ability to absorb light is
one the main reasons it is so useful in industrial and medical
applications," said Wei-Qiang Han, a scientist at Brookhaven's Center
for Functional Nanomaterials (CFN) and lead author on both papers. It
is used as a photocatalyst for converting sunlight to electricity in
solar cells and also has applications in the production of hydrogen,
in gas sensors, in batteries, and in using sunlight to degrade some
environmental contaminants. It is also a common ingredient in
sunscreen.
Many scientists have explored ways to improve the light-absorbing
capability of titanium oxide, for example, by "doping" the material
with added metals. Han and his coworkers took a new approach. They
enhanced the material's light-absorption capability by simply
introducing nanocavities, completely enclosed pockets measuring
billionths of a meter within the 100-nanometer-diameter solid titanium
oxide rods.
The resulting nanocavity-filled titanium oxide nanorods were 25
percent more efficient at absorbing certain wavelengths of ultraviolet
A (UVA) and ultraviolet B (UVB) solar radiation than titanium oxide
without nanocavities.
"Our research demonstrates that titanium oxide nanorods with
nanocavities can dramatically improve the absorption of UVA and UVB
solar radiation, and thus are ideal new materials for sunscreen," Han
said.
The cavity-filled nanorods could also improve the efficiency of
photovoltaic solar cells and be used as catalysts for splitting water
and also in the water-gas-shift reaction to produce pure hydrogen gas
from carbon monoxide and water.
The method for making the cavity-filled rods is simple, says Han. "We
simply heat titanate nanorods in air. This process evaporates water,
transforming titanate to titanium oxide, leaving very densely spaced,
regular, polyhedral nanoholes inside the titanium oxide."
In the second paper, Han and his collaborators describe a new
synthesis method to make iron-doped titanate nanotubes, hollow tubes
measuring approximately 10 nanometers in diameter and up to one
micrometer (one millionth of a meter) long. These experiments were
also aimed at improving the material's photoreactivity. The scientists
demonstrated that the resulting nanotubes exhibited noticeable
reactivity in the water-gas-shift reaction.
"Although the activity of the iron-doped nanotubes was not as good as
that of titanium oxide loaded with metals such as platinum and
palladium, the activity we observed is still remarkable considering
that iron is a much less expensive metal and its concentration in our
samples was less than one percent," Han said.
The scientists also observed interesting magnetic properties in the
iron-doped nanotubes, and will follow up with future studies aimed at
understanding this phenomenon.
Materials developed in these studies were analyzed using several of
Brookhaven Lab's unique tools and methods for the characterization of
nanostructures, including transmission electron microscopy and various
techniques using x-ray and infrared beams at the Lab's National
Synchrotron Light Source (NSLS).
This research, which has clear connections to improved energy
technologies, was funded by the Office of Basic Energy Sciences within
the U.S. Department of Energy's Office of Science.
Collaborators on the Advanced Materials paper include Lijun Wu, Robert
F. Klie, and Yimei Zhu, all of Brookhaven's Center for Functional
Nanomaterials (CFN). For the Journal of Physical Chemistry paper,
collaborators include Brookhaven chemists Wen Wen and Jonathan Hanson;
Ding Yi, Mathew Maye, and Oleg Gang of the CFN; Zhenxian Liu of the
Carnegie Institution of Washington; and Laura Lewis, formerly at the
CFN and now at Northeastern University.
|