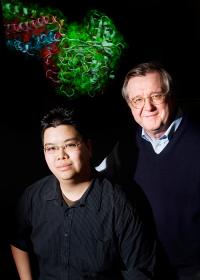
|
Illinois graduate research assistant Eric Lee
(left) and physics professor Klaus Schulten used steered molecular
dynamics to model the behavior of every atom of the fibrinogen
molecule as it was stretched. The computation involved over a
million atoms, and required six months to complete.
Photo by L. Brian Stauffer, U. of I. News
Bureau. |
Fibrinogen is highly elastic, able to
reversibly stretch to two or three times its original length.
�Once they�re formed, blood clots have to be elastic because they have
a mechanical function to withstand blood pressure,� said Klaus
Schulten, holder of the Swanlund Chair in Physics at Illinois.
Understanding what gives fibrinogen its flexibility could help in the
design of drugs to enhance their function, he said.
�We investigated what makes blood clots elastic,� said Eric Lee, a
graduate research assistant and student in the M.D./Ph.D. program at
Illinois. �How do we make them easier to break up or make them less
likely to rupture"�
Bernard Lim, a cardiologist at Mayo and an expert on the science of
blood clots, contacted Schulten�s group in 2006 for help with a
puzzling finding. Lim had conducted a series of experiments using
atomic force microscopy to measure the amount of force required to
stretch individual fibrinogen molecules.
After dozens of trials, Lim had come up with a �force extension curve�
that showed how the fibrinogen molecule behaved when it was stretched.
His data indicated that the fibrinogen molecule elongates in a
sequential fashion, with three distinct phases. But he could not tell
which parts of the fibrinogen molecule were involved.
Fibrinogen is a symmetrical molecule, containing a central region
connected to two end regions by long, interweaving coiled chains,
called alpha helices. These �coiled coils� were believed to give the
molecule its elasticity. But how"
The Illinois team used a computational approach to tackle the mystery.
Using steered molecular dynamics (SMD), they modeled the behavior of
every atom of the fibrinogen molecule as it was stretched. The
computation involved more than a million atoms, and required six
months to complete.
The resulting simulation (see below) generated
a force extension curve that matched the one Lim had produced.
�This was an incredibly strong piece of evidence that what (Lim) saw
wasn�t just in the eye of the beholder, but he saw really a property
of the protein,� Schulten said.
The simulation also showed in molecular detail how the fibrinogen
molecule responded to stretching. Each phase in the force extension
curve corresponded directly with a distinct set of events in the
elongation of the molecule.
�The simulations revealed that � the extension occurs in a specific
and orderly pattern, with distinct regions within the coiled-coil
unraveling before others,� the authors wrote.
Lim had also demonstrated that changes in calcium levels or in the pH
(acidity) of a blood clot could alter fibrinogen elasticity, a finding
that could influence the design of pharmaceutical agents.
�By understanding what happens at the molecular level, you can
understand where to target drugs,� Lee said.
This study points to the efficacy of combining molecular dynamics
simulations with experimental data on actual molecules, Schulten said.
This is proving to be an effective way to get to the heart of
molecular behavior, he said.
Simulations can test important, but potentially ambiguous,
experimental findings, Schulten said. �And we can see (the behavior of
the molecule) in chemical detail, in atomic detail. We see the full
chemistry of this mechanical process.�
|