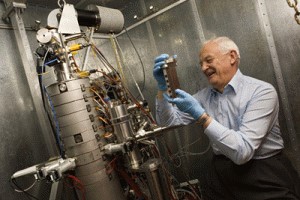
John Silcox, the David E. Burr Professor of
Engineering, with the new scanning transmission electron
microscope. The new instrument gives materials scientists
unprecedented atomic level views of their work.
Lindsay France/University Photography
|
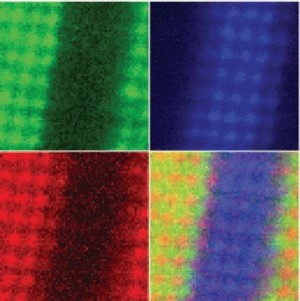
Electron spectroscopy identifies
atoms in a cross section of an artificially layered material.
Upper left shows an edge between layers of lanthanum and manganese
atoms; upper right, an edge between lanthanum and titanium; lower
left, a manganese-lanthanum edge; lower right, all three images
combined. A line of purple shows that manganese and titanium atoms
are mixing across their boundary, a problem that needs to be fixed
to make the material useful.
Image � by
Robert Muller |
The instrument is a new type of scanning
transmission electron microscope (STEM), built by the NION Company of
Kirkland, Wash., under an instrument-development award to Cornell from
the National Science Foundation (NSF). John Silcox, the David E. Burr
Professor of Engineering at Cornell, and Ondrej Krivanek of NION are
co-principal investigators on the project.
The microscope incorporates new aberration-correction technology
designed by Krivanek that focuses a beam of electrons on a spot
smaller than a single atom - more sharply and with greater intensity
than previously possible. This allows information previously hidden in
the background, or "noise," to be seen. It also provides up to a
hundredfold increase in imaging speed.
The capabilities of the new instrument in analyzing a test sample are
described in an article in the Feb. 22, 2008,
issue of the journal Science by Muller, Silcox, Krivanek and
colleagues at Cornell and in Korea and Japan.
It allows scientists to peer inside a material or a device and see how
it is put together at the atomic scale where quantum effects dominate
and everyday intuition fails. One of the most important applications
of the new instrument will be to conduct what Silcox calls "materials
pathology" to aid researchers in their development of new materials to
use in electronic circuits, computer memories and other nanoscale
devices. "We can look at structures people have built and tell them if
they've built what they thought they did," Silcox explained.
A STEM shoots an electron beam through a thin-film sample and scans
the beam across the sample in subatomic steps. In addition to forming
an image, the new microscope can identify atoms in its path by a
process called electron energy-loss spectrometry. Atoms in the path of
the beam absorb energy from some of its electrons to kick their own
electrons into higher orbits. The amount of energy this takes is
different for each kind of atom.
The detector that collects electrons emerging from the sample measures
the energy losses, and from this the atoms in the path of the beam can
be identified. The detector can simultaneously produce multiple images
-- one for every different species of atom in the sample, and these
can be color-coded, each color representing a different electron
energy signature.
The method also can show how atoms are bonded to one another in a
crystal, because the bonding creates small shifts in the energy
signatures. In earlier STEMS, many electrons from the beam, including
those with changed energies, were scattered at wide angles by simple
collisions with atoms. The new STEM includes magnetic lenses that
collect emerging electrons over a wider angle. Previously, Silcox said,
about 8 percent of the emerging electrons were collected, but the new
detector collects about 80 percent, allowing more accurate readings of
the small changes in energy levels that reveal bonding between atoms.
More complete collection and a brighter and a more sharply focused
beam also allow the new microscope to scan much faster. In early tests
it collected a 4,096-pixel image in about 30 seconds, 50 to 100 times
faster than in conventional STEMs.
To demonstrate the capability of the new instrument, Muller examined a
sample consisting of layers of two different materials:
lanthanum-strontium-manganese oxide and strontium-titanate. This was
done as part of a research project on which he is collaborating with
scientists in Korea and Japan. "It's an artificial structure that will
have interesting magnetic and electrical properties," he said, "but
for it to work properly we have to make atomically sharp interfaces
between the layers. It's really important to know if a few atoms
leaked across the interface."
In the color image from the new STEM, where manganese appears red and
titanium blue, a line of purple shows mixing at the edge between the
two layers. "We've learned that there's room for improvement," Muller
says, adding "This wasn't our best sample, but if we had put that one
in it would have been a fairly boring image."
The new instrument arrived at Cornell in October, and is still
undergoing calibration and testing.
The problems that limited electron imaging were identified as long ago
as 1935, Silcox said, and ideas for overcoming them were outlined in
1947. But it was not until very recently that the engineering
obstacles to putting them into practice were overcome. Largely, he
said, this is because the problem required advanced computing,
including computers to design the instrument, computer-controlled
machinery to manufacture parts to fine tolerances, and computers to
control the instrument itself.
|