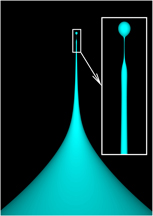
This image, created using a modeling technique
called finite element computation, shows a droplet forming from a
liquid that has been exposed to an electric field. Chemical
engineers at Purdue are the first to mathematically describe
precisely how the process forms droplets, an advance that could
have applications in areas ranging from manufacturing to medical
diagnostics.
� Purdue University, School of Chemical Engineering
|
New findings showed that a liquid's viscosity
plays a vital role in drop formation and size, a discovery that
contradicts conventional wisdom, Basaran said.
The researchers first created simulations to describe droplet
formation mathematically, and then they performed experiments to
support the computational work.
"Computational simulations are now making it possible to understand
such phenomena," he said. "But you always want to back up simulations
with experimental data if at all possible."
The findings are detailed in a paper appeared
in the January 2008 issue of Nature Physics.
The paper was written by doctoral student Robert T. Collins,
undergraduate student Jeremy J. Jones, professor Michael T. Harris,
and Basaran, all in the School of Chemical Engineering.
Researchers have known for decades that applying an electric field to
liquid drops causes the formation of structures that have a perfect
cone at the leading edge.
"Each drop takes on the shape of a chocolate kiss," Basaran said.
Then, a thin ribbonlike strand of fluid is emitted from the leading
edge of the droplet and breaks up into smaller droplets.
"This was discovered about a century ago," Basaran said. "Nobody could
really show precisely how it happened, but technologically it became
very important."
The method could make possible future technologies for creating
flexible electronic circuits and solar cells by spraying material in
ultra-thin layers.
"Making small drops and controlling drop size is a big deal, and there
are many techniques that people are working on to be able to model
these computationally or theoretically," Basaran said. "There are many
applications that would benefit by knowing the drop size. You cannot
predict the drop sizes unless you have simulations to tell you how the
strand is going to develop and break up into little droplets."
The same phenomenon occurs in rain clouds. As rain droplets pick up an
electrical charge, they take on an elongated football shape. Thin
strands form at each end of the football, and those strands, in turn,
form small droplets.
"Again, the ends shoot these little strands or jets, which break up
into drops," Basaran said. "And this has been suspected for a century
to play a major role in how thunderstorms work."
Understanding how the drops form is difficult because the strands are
many times thinner than the original liquid from which they formed,
which makes the mathematics especially challenging.
"Others had not solved these equations in their full form before
because they are very difficult, and we have now solved them without
any approximations," Basaran said.
Conventional modeling methods use "diffuse interface" techniques,
which do not precisely predict how the strands and droplets form, he
said.
The Purdue researchers used a more precise method called finite
elements with elliptic mesh generation. The technique breaks down a
material into many small segments and solves the mathematical
equations governing the behavior of each segment separately. Using the
method enables researchers to understand the dynamic, changing shapes
of each segment making up the drop-forming strands and the droplets.
The technique allowed the engineers to negotiate the dramatic size
differences between the strands and original liquid, a process that
falls in the realm of "multi-scale modeling."
"Our approach allowed us to do this multi-scale modeling in one big
calculation," Basaran said.
In the experiments, an electric voltage was applied to a small metal
rod positioned about an inch from a puddle of liquid. The liquid was
pulled toward the tip of the rod and took on a conical appearance.
Strands of liquid, which subsequently broke into droplets, were then
emitted from the liquid cone.
The Purdue researchers conducted experiments using liquids with large
viscosities, including those similar to fuels, whereas past research
has concentrated on lower viscosity fluids like water.
"This may prove to be important in combustion applications because
fuel is sprayed into engine cylinders using fuel injectors," Basaran
said. "We studied higher viscosity fluids to see the effect of
viscosity, which other people had never seen before. It turns out that
the viscosity actually has a big effect on droplet size."
Future research will continue to study how droplet formation is
influenced by other characteristics of a liquid. These characteristics
include the electrical conductivity of fluids and the surface tension,
the latter being responsible for the beading of water droplets on a
recently waxed car.
"We are just scratching the surface because there is such a large
range of viscosities and other characteristics of fluids that affect
droplet formation and size," Basaran said. "There could be many
surprises."
The research has been funded by the U.S. Department of Energy.
|