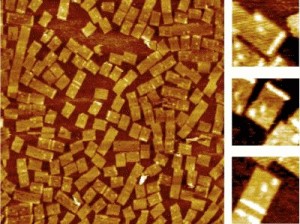
On the left is an AFM image of DNA nanoarrays bound
to their RNA targets at 1500nm x 1500nm scale. The zoom-in images
(150nm x 150nm scale) on the right clearly show the barcode (white
dots) that identifies the nanoarray and the RNA hybridization
signal on the DNA nanoarray ( white bar).
Image by Yonggang Ke
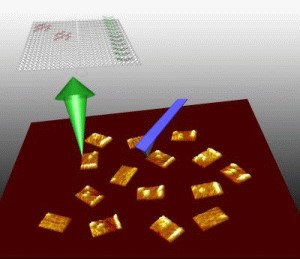
By controlling the exact position and location of
the chemical bases within a synthetic replica of DNA, Yan
programmed a single stranded genomic DNA, M13, into nanotiles to
contain the probes for specific gene expression targets. On the
surface of each DNA probe tile is a dangling single stranded piece
of DNA that can bind to the RNA target of interest. Each probe
actually contains two half probes, so when the target RNA comes
in, it will hybridize to the half probes and turn the single
stranded dangling probes into a stiff structure. The group uses a
powerful instrument, atomic force microscopy (AFM), which allows
the researchers to image the tiles at the single molecule level.
After binding to the DNA probe, the DNA-RNA hybridization becomes
stiffened, it can be sensed by the atomic force microscope
cantilever (blue arm), shown by bright line on the DNA tile, which
is due to a height increase. The result is a mechanical,
label-free detection of RNA.
Image by Hao Yan
|
Yan led an interdisciplinary ASU team to
develop a way to use structural DNA nanotechnology to target the
chemical messengers of genes, called RNA.
The team included: lead author and chemistry and biochemistry graduate
student Yonggang Ke; assistant professor of chemistry and biochemistry
Yan Liu; Center for Single Molecule Biophysics director and physics
professor Stuart Lindsay; and associate professor in the School of
Life Sciences, Yung Chang.
"This is one of the first practical applications of a powerful
technology, that, till now, has mainly been the subject of research
demonstrations,� said Lindsay. �The field of structural DNA
nanotechnology has recently seen much exciting progress from
constructing geometrical and topological nanostructures through tile
based DNA self-assembly initially demonstrated by Ned Seeman, Erik
Winfree and colleagues,� said Yan.
A recent breakthrough of making spatially addressable DNA nanoarrays
came from Paul Rothemund�s work on scaffolded DNA origami, a method in
which a long, single-stranded viral DNA scaffold can be folded and
stapled by a large number of short synthetic �helper strands� into
nanostructures that display complex patterns.
�But the potential of structural DNA nanotechnology in biological
applications has been underestimated, and if we look at the process of
DNA self-assembly, you will be amazed that trillions of DNA
nanostructures can form simultaneously in a solution of few
microliters, and very importantly, they are biocompatible and water
soluble,� said Yan.
DNA chip and microarray technology have become
a multi-billion dollar industry as scientists use it to examine
thousands of genes at the same time for mutations or uncovering clues
to disease. However, because DNA probes are pinned to the solid
surface of the microarray chips, it is relatively slow process for the
targets to search and find the probes. Also, it is hard to control the
distances between the probes with nanometer accuracy.
�In this work, we developed a water soluble nanoarray that can take
advantage of the DNA self-assembling process and also have benefits
that the macroscopic DNA microchip arrays do not have,� said Yan. �The
arrays themselves are reagents, instead of solid surface chips.�
To make the DNA origami RNA probes, Yan has taken advantage of the
basic DNA pairing rules in the DNA chemical alphabet (�A� can only
form a zipper-like chemical bond with �T� and �G� only pair with �C�).
By controlling the exact position and location of the chemical bases
within a synthetic replica of DNA, Yan programmed a single stranded
genomic DNA, M13, into nanotiles to contain the probes for specific
gene expression targets.
Yan refers to the self-assembled DNA nanoarrays as nucleic acid probe
tiles, which look like a nanosized postage stamp. In a single step,
the M13 scaffold system can churn out as many as 100 trillion of the
tiles with close to100 percent yield.
Yan�s team designed three different DNA probe tiles to detect three
different RNA genes along with a bar code index to tell the tiles
apart from each other. �Each probe can be distinguished by its own bar
code, so we mixed them together in one solution and we used this for
multiplex detection,� said Yan. The group uses a powerful instrument,
atomic force microscopy (AFM), which allows the researchers to image
the tiles at the single molecule level.
On the surface of each DNA probe tile is a dangling single stranded
piece of DNA that can bind to the RNA target of interest. �Each probe
actually contains two half probes, so when the target RNA comes in, it
will hybridize to the half probes and turn the single stranded
dangling probes into a stiff structure,� said Yan. �When it is
stiffened, it will be sensed by the atomic force microscope cantilever,
and you can see a bright line, which is a height increase. The result
is a mechanical, label-free detection.�
The technology is able to detect minute quantities of RNA. �Since the
DNA-RNA hybridization has such a strong affinity, in principle, a
single molecule would be able to hybridize to the probe tile,� said
Yan.
Although there are still many technical hurdles yet to overcome, the
group is excited about the potential applications of the technology.
�What our approach provides is that the probe tiles are a
water-soluble reagent, so the sample volume can potentially be shrunk
down to the volume of a single cell level. Our ultimate goal is to
detect RNA gene expression at the single cell level.�
The research was performed in the Biodesign Institute�s Center for
Single Molecule Biophysics, Center for Infectious Diseases and
Vaccinology, and ASU�s Department of Chemistry and Biochemistry,
Department of Physics and School of Life Sciences.
This research is partly supported by funding from NIH and from NSF,
U.S. Air Force Office of Scientific Research, and Office of Naval
Research.
|