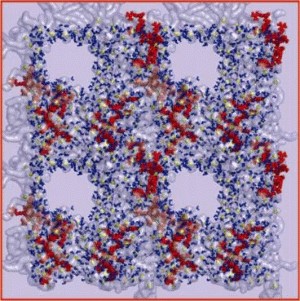
Special HIV peptide interacts with a cell membrane
to open a hole in the cell, offering scientists a new pathway for
delivering materials to a cell.
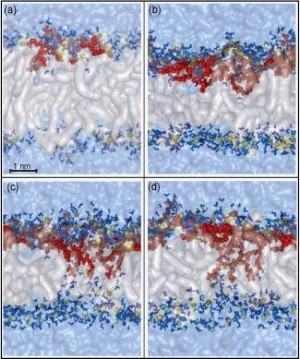
A computer generated image of a cell membrane being
"pierced" by HIV peptides. The positively charged HIV peptides (shown
in red) are drawn to negatively charged phosphates (shown in
yellow) in the cell membrane. When an HIV peptide cannot satisfy
itself with the negative charges available on the cell membrane
surface it is directly attached to, it reaches through the
membrane to grab negatively charged phosphates on the other side,
opening a hole in the cell.
Images by Rensselaer Polytechnic Institute/Angel
Garcia
|
For the last decade, scientists have known
that a positively charged, 11-amino-acid chain of HIV (HIV-1 Tat
protein) can do the nearly unthinkable �X cross through the cell
membrane. Sometimes referred to as an "arrow protein," HIV-1 Tat
pierces the cell membrane and carries a cargo though the cell membrane.
Its unique cell-puncturing ability has been the subject of hundreds of
scientific articles investigating the type of materials that can
piggyback on the peptide and also enter the cell. Researchers have
proposed using the peptide to deliver genes for gene therapy and drugs
that need to be delivered directly to a cell. But despite many
potential medical applications, the actual mechanism that opens the
holes in the cell remained undiscovered.
The Rensselaer researchers have discovered that the positively charged
HIV peptide is drawn to negatively charged groups inside the cell
membrane. When the HIV peptide cannot satisfy itself with the negative
charges available on the cell membrane surface it is directly attached
to, it reaches through the membrane to grab negatively charged groups
in the molecules on the other side, opening a transient hole in the
cell.
"What we saw in our computer calculations wasn't at all what we
expected to see when we began," said co-lead author and Senior
Constellation Professor of Biocomputation and Bioinformatics Angel
Garcia. "The mechanism for entrance in the cell was clear in one
simulation, but in some instances simulations show one result and you
never see that result again. Then we started doing other simulations
and it kept happening again and again."
Garcia and his collaborator, postdoctoral researcher Henry Herce,
initially set out to uncover how the peptide interacts with a lipid
bilayer that is used to model the cell membrane. A highly efficient
biological system, the cell membrane is composed of a lipid bilayer (made
up of two monolayers) designed to protect the cell by preventing the
influx of material. Each lipid in the bilayer has a polar, or charged,
end and a non-polar end. A monolayer of lipids faces the exterior of
the cell, with the polar end facing the outside of the cell. Another
monolayer is under the first layer, forming the bilayer. The polar end
of the lower layer faces the interior of the cell, forming a middle
section containing the uncharged halves of both monolayers.
Because charged particles seek each other in order to neutralize
themselves and achieve a more stable state, the surface of the polar
cell membrane and the positively charged HIV peptide are drawn to one
another. But the interior of the bilayer is not charged and forms a
strong barrier against the entrance of any charged material.
As was expected, in their simulations the researchers observed that
the positive charges in the peptide quickly attached to the surface of
the cell membrane and sought out and reacted with negatively charged
phosphates from the charged portion of the lipid bilayer to satisfy
their need for neutrality. "Then the peptide entered the forbidden
territory of the cell," Garcia said. The positively charged peptide
entered the membrane. "This is when this mechanism starts to challenge
conventional wisdom," he said.
The researchers' model systems show the peptides grabbing for
surrounding negative charges, but when no more of those charges are
available due to their greedy peptide neighbors, some of the peptides
reach into the cell membrane and grab negative charged phosphates from
the other side. This opens a hole in the cell membrane and allows the
flow of water and other material into the cell. Once all the peptides
have been neutralized, the reaction stops and the hole closes, leaving
behind a healthy, viable cell.
For the paper, the researchers reported a dozen different simulations
run through a high-powered cluster of computers. Each simulation
required a long process of testing and validating results. Garcia's
computer cluster is now running simulations on the use of
antimicrobial proteins which will open a pore in the cell and keep it
open, killing the cell. Antimicrobial proteins have promising direct
applications for killing harmful cells in the body.
Garcia hopes to harness the power of Rensselaer's newly opened
Computational Center for Nanotechnology Innovations (CCNI), which
houses the world's most powerful university-based supercomputing
center. The CCNI will allow him to compile two years' worth of data on
his normal cluster in just 10 to 20 days.
The research was funded by the National Science Foundation (NSF)
through the Rensselaer Nanoscale Science and Engineering Center for
Directed Assembly of Nanostructures (NSEC) and Rensselaer Polytechnic
Institute. Garcia is a member of the Rensselaer Center for
Biotechnology and Interdisciplinary Studies.
|