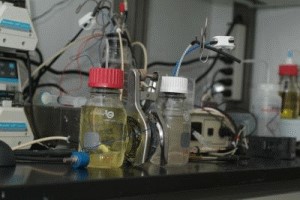
The microbial fuel cell (MFC), shown in this
tabletop setup, can take common sources of organic waste such as
human sewage, animal waste, or agricultural runoff and convert
them into electricity.
Credit: The Biodesign Institute at Arizona State
University
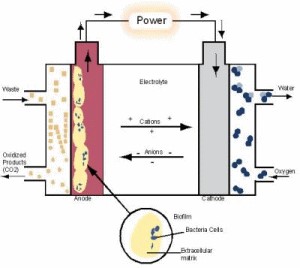
Bacteria have evolved to utilize almost any
chemical as a food source. In the microbial fuel cell, bacteria
form a biofilm, a living community that is attached to the
electrode by a sticky sugar and protein coated biofilm matrix.
When grown without oxygen, the byproducts of bacterial metabolism
of waste include carbon dioxide, electrons and hydrogen ions.
Electrons produced by the bacteria are shuttled onto the electrode
by the biofilm matrix, creating a thriving ecosystem called the
biofilm anode and generating electricity.
Credit: Janelle Curtis, Biodesign Institute at
Arizona State University
|
"There is a lot of biomass out there that we
look at simply as energy stored in the wrong place," said Bruce
Rittmann, director of the center. "We can take this waste, keeping it
in its normal liquid form, but allowing the bacteria to convert the
energy value to our society's most useful form, electricity. They get
food while we get electricity."
Waste not
Bacteria have such a rich diversity that researchers can find a
bacterium that can handle almost any waste compound in their daily
diet. By linking bacterial metabolism directly with electricity
production, the MFC eliminates the extra steps necessary in other fuel
cell technologies. "We like to work with bacteria, because bacteria
provide a cheap source of electricity," said Marcus.
There are many types of MFC reactors and research teams throughout the
world (http://microbialfuelcell.org). However, all reactors share the
same operating principles. All MFCs have a pair of battery-like
terminals: an anode and cathode electrode. The electrodes are
connected by an external circuit and an electrolyte solution to help
conduct electricity. The difference in voltage between the anode and
cathode, along with the electron flow in the circuit, generate
electrical power.
In the first step of the MFC, an anode respiring bacterium breaks down
the organic waste to carbon dioxide and transfers the electrons
released to the anode. Next, the electrons travel from the anode,
through an external circuit to generate electrical energy. Finally,
the electrons complete the circuit by traveling to the cathode, where
they are taken up by oxygen and hydrogen ions to form water.
What is the matrix?
"We knew that the MFC process is relatively stable, but one of the
biggest questions is: How do the bacteria get the electrons to the
anode?" said Marcus. The bacteria depend on the anode for life. The
bacteria at the anode breathe the anode, much like people breathe air,
by transferring electrons to the anode. Because bacteria use the anode
in their metabolism, they strategically position themselves on the
anode surface to form a bacterial community called a biofilm.
Bacteria in the biofilm produce a matrix of material so that they
stick to the anode. The biofilm matrix is rich with material that can
potentially transport electrons. The sticky biofilm matrix is made up
of a complex of extracellular proteins, sugars, and bacterial cells.
The matrix also has been shown to contain tiny conductive nanowires
that may help facilitate electron conduction.
"Our numerical model develops and supports the idea that the bacterial
matrix is conductive," said Marcus. In electronics, conductors are
most commonly made of materials like copper that make it easier for a
current to flow through . "In a conductive matrix, the movement of
electrons is driven by the change in the electrical potential." Like a
waterfall, the resulting voltage drop in the electrical potential
pushes the flow of electrons.
The treatment of the biofilm matrix as a conductor allowed the team to
describe the transport of electrons driven by the gradient in the
electrical potential. The relationship between the biofilm matrix and
the anode could now be described by a standard equation for an
electrical circuit, Ohm's law.
Within the MFC is a complex ecosystem where bacteria are living within
a self-generated matrix that conducts the electrons. "The whole
biofilm is acting like the anode itself, a living electrode," said
Marcus. "This is why we call it the 'biofilm anode.'"
Life at the Jolt
The concept of the 'biofilm anode' allowed the team to describe the
transport of electrons from bacteria to the electrode and the
electrical potential gradient. The importance of electrical potential
is well known in a traditional fuel cell, but its relevance to
bacterial metabolism has been less clear. The next important concept
the team had to develop was to understand the response of bacteria to
the electrical potential within the biofilm matrix.
Bacteria will grow as long as there is an abundant supply of nutrients.
Jacques Monod, one of the founding fathers of molecular biology,
developed an equation to describe this relationship. While the team
recognized the importance of the Monod equation for bacteria bathed in
a rich nutrient broth, the challenge was to apply the Monod equation
to the anode, a solid.
Previous studies have shown that the rate of bacterial metabolism at
the anode increases when the electrical potential of the anode
increases. The researchers could now think of the electrical potential
as fulfilling the same role as a bacterial nutrient broth. The team
recognized that the electrical potential is equivalent to the
concentration of electrons; and the electrons are precisely what the
bacteria transfer to the anode.
Equipped with this key insight, the team developed a new model, the
Nernst-Monod equation, to describe the rate of bacterial metabolism in
response to the "concentration of electrons" or the electrical
potential.
Promise meeting potential
In their model, the team identified three crucial variables to
controlling an MFC: the amount of waste material (fuel), the
accumulation of biomass on the anode, and the electrical potential in
the biofilm anode. The third factor is a totally novel concept in MFC
research.
"Modeling the potential in the biofilm anode, we now have a handle on
how the MFC is working and why. We can predict how much voltage we get
and how to maximize the power output by tweaking the various factors,"
said Marcus. For example, the team has shown that the biofilm produces
more current when the biofilm thickness is at a happy medium, not too
thick or thin.
"If the biofilm is too thick," said Marcus, "the electrons have to
travel too far to get to the anode. On the other hand, if the biofilm
is too thin, it has too few bacteria to extract the electrons rapidly
from the fuel."
To harvest the benefits of MFCs, the research team is using its
innovative model to optimize performance and power output. The project,
which has been funded by NASA and industrial partners OpenCEL and
NZLegacy, lays out the framework for MFC research and development to
pursue commercialization of the technology.
|