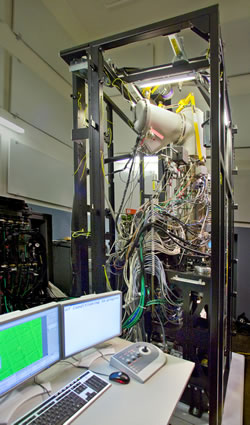
TEAM 0.5, the world's best transmission electron
microscope, is being assembled at the National Center for Electron
Microscopy.
Photo: Roy Kaltschmidt, Berkeley Lab CSO
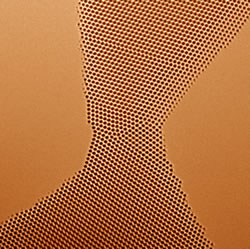
Where these two gold crystals meet they are joined
by a complex arrangement of atoms, forming a nanobridge that
accommodates their different orientations. The gold atoms are 2.3
angstroms apart. TEAM 0.5's unprecedented signal-to-noise ratio
makes it possible to distinguish individual atoms and, at the
edges of the two crystals, deduce their position in three
dimensions.
|
Now that TEAM 0.5's basic systems are
operational, additional components and facilities are being completed
and tuned, including a state-of-the-art control room display that
shows the sample under the microscope on a flat panel resembling a
wide-screen, high-definition TV. After a long series of rigorous tests
and adjustments, TEAM 0.5 will become available to outside users by
October, 2008.
Atom by atom in 3-D
In preliminary tests at the FEI Company, before the TEAM 0.5 was
shipped, NCEM's Christian Kisielowski tested the microscope's ability
to resolve individual atoms and precisely locate their positions in
three dimensions. He made a series of images of two gold crystals
connected by a "nanobridge" only a few dozen atoms wide. From each
exposure to the next, individual gold atoms could be seen changing
positions.
To achieve this extraordinary resolution, TEAM 0.5 embodies technical
advances that have only recently become possible, including
ultra-stable electronics, improved aberration correctors, and an
extremely bright electron source.
Spherical aberration degrades images, making points of light look like
disks, and correcting it can make dramatic improvements to image
resolution. (This was famously demonstrated in 1993, when spherical
aberration in the Hubble Space Telescope's optical lenses was
corrected in a special space mission.) In the case of electron
microscopes, a series of multipole magnetic lenses of varying
geometries shapes the electron beam.
"Correcting spherical aberration in an electron microscope has long
been possible in theory," says Dahmen. "But only recently has it
become practical, because today's stable electronics reduce drift and
fast computers allow continuous adjustments in real time." Corrector
technology has even become available commercially, says Dahmen, "but
no off-the-shelf corrector can match TEAM 0.5's ability to compensate
even higher-order aberrations."
Correcting spherical aberration makes it possible to use the TEAM 0.5
not only for broad-beam, "wide-angle" images but also for scanning
transmission electron microscopy (STEM), in which the tightly focused
electron beam is moved across the sample as a probe, capable of
performing spectroscopy on one atom at a time -
an ideal way to precisely locate impurities in an otherwise
homogeneous sample, such as individual dopant atoms in a semiconductor
material.
Aberration correction is also essential for another advanced feature
of TEAM 0.5: its ability to maintain high resolution with lower
electron beam energies.
"Low energy electrons have longer wavelengths, so they are harder to
focus," Dahmen explains. "Aberration correction allows better than
one-angstrom resolution with excellent contrast even at 80 kilovolts.
This is important when you don't want to damage the sample with a
high-energy beam - in biological studies, for
example."
It's not just high resolution that makes TEAM 0.5 the world's best
microscope, Dahmen says. When all the electrons in the beam focus at
the same plane, image contrast and signal-to-noise ratio improve
tremendously.
"It's because the signal-to-noise ratio is so good that you can adjust
focus atom by atom, with enough sensitivity to obtain information
about the three-dimensional atomic structure of a single nanoparticle."
Dahmen adds, "This brings us within reach of meeting the great
challenge posed by the famous physicist Richard Feynman in 1959: the
ability to analyze any chemical substance simply by looking to see
where the atoms are."
The position of individual atoms in a structure can be determined by
taking images at different angles, from which the computer
reconstructs a 3-D tomograph of the sample, as in a CAT scan. To make
this possible an innovative system capable of tilting and rotating the
sample, and moving it up, down, or sideways under the electron beam,
is also being developed at NCEM.
Much smaller than sample stages now in use, the new TEAM stage will be
housed entirely inside the microscope column. Manipulating the sample
by such methods as minute piezoelectric "crawlers" that change shape
when electricity is applied, the new stage will be able to control and
reproduce the sample's position and attitude with an accuracy of less
than a billionth of a meter.
Installation of the new stage must await the next phase of the TEAM
Project: the TEAM I microscope, due to be set up at NCEM early in
2009.
While TEAM 0.5 corrects spherical aberration in both the "probe" beam
(the electron beam before it strikes the sample) and the image beam (after
it exits the sample, but before it reaches the detector), TEAM I will
also correct chromatic aberration in the image beam, which has never
beeen accomplished before. Spherical aberration is caused by the shape
of a lens; chromatic aberration results when a lens refracts light or
electrons of different wavelengths (different colors or energies) at
different angles.
"Correcting chromatic aberration is harder and takes more space," says
Dahmen. "The chromatic aberration corrector will add two feet to the
height of the TEAM I column. But the new configuration will also allow
us to enlarge the gap between the pole pieces, into which the sample
fits. In TEAM 0.5 this gap is only about two millimeters, so we have
to use traditional outside-mounted sample stages, with limited space
to manipulate the sample. In TEAM I the gap will be five millimeters;
the sample stage will have much greater freedom of movement."
New vistas in the realm of the small
TEAM 0.5 and TEAM I will be housed side by side at NCEM for some time,
occupying the two multistory "silos" that until recently were the
homes of the historic High-Voltage Electron Microscope and the Atomic
Resolution Microscope, the most powerful microscopes in the world when
NCEM was established in the early 1980s.
Ambitious as those microscopes were in their day, says TEAM's Project
Manager, Peter Denes of the Engineering Division, "when the TEAM
Project was launched in 2004, it was not quite clear if the goals
could even be achieved. The electron microscopy community had never
done a collaborative project like TEAM before, and certainly not with
full DOE project-management rigor."
Says Denes, "Perhaps the biggest contributor to success was a series
of scientific workshops that contributed to forming a converging
opinion on what the next steps would be, and what would constitute
success. That helped in getting everyone - if
not quite on the same page - at least in the
same book."
Dahmen agrees. "This is a big jump for the microscopy community.
TEAM's success will open the door to other ambitious developments
around the world."
Dahmen suggests at least two broad categories of researchers who will
benefit from the powerful new electron microscopes: experts with
sophisticated microscopy problems to solve, and scientists less
familiar with electron microscopy but with a particular problem for
which microscopy can provide the answer.
"For example, Jim Zuo at the University of Illinois is doing studies
of electron diffraction from the surface of single nanoparticles,"
Dahmen says. "He sees evidence of surface contraction. But when we at
NCEM do imaging of similar nanoparticles, we find that the surface is
expanding. Jim looks forward to using the TEAM microscope because it
can do diffraction and imaging of the same particle at the same time
- a grand experiment, and the only way to solve
the apparent contradiction."
An example of a problem-solving nonspecialist, says Dahmen, might be a
materials scientist who has created a new kind of nanostructure, such
as a tetrapod semiconductor, and needs to know exactly where in this
complex, three-dimensional shape the impurity atoms reside. "TEAM's
ability to image the structure in 3-D through tomography and its
ability to do spectroscopy with single-atom sensitivity can identify
each kind of atom at each position in the structure. That has never
been possible before."
The basic TEAM components of aberration correction, enhanced
signal-to-noise ratio, single-atom sensitivity, and an ultrabright
beam that can be used in both TEM and STEM modes -
all the while manipulating the sample in the beam -
are goals that until recently seemed at the very edge of technological
daring. All are on track, and some have been solved ahead of schedule.
The TEAM Project's continuing success, signaled by the installation of
TEAM 0.5 at NCEM, has opened the possibility of numerous future
advances in electron microscopy that were barely conceivable when TEAM
was launched.
The multi-institutional TEAM project represents a new kind of
distributed planning and cooperation for the electron microscope
community, moving beyond the limited, incremental improvements of
individual investigators and harnessing the power of collaboration.
Argonne National Laboratory is leading the development of the
chromatic-aberration corrector in close collaboration with CEOS in
Heidelberg. The University of Illinois's Frederick Seitz Materials
Laboratory is jointly developing the new piezoelectric-controlled
sample stage with Berkeley Lab's NCEM, and Oak Ridge National
Laboratory is helping to optimize the new probe corrector. NCEM acts
as project leader to integrate the individual components into single
instruments, in close collaboration with all other TEAM partners. The
TEAM Project is supported by the U.S. Department of Energy's Office of
Science.
|