|
Each of these jars contains the same substance. The difference is the size of the particles. Quantum dots, suspended in liquid, absorb white light and then reemit it in a specific color that depends on the particle's size. Each quantum dot is about one ten-millionth of an inch in diameter and is composed of a few hundred atoms of material.
Image by Xiaohu Gao, University of Washington
|
|
A fluorescent image of the cell taken 15 minutes after introducing the quantum dot-siRNA complex. At this early stage the particles are in the cell membrane.
|
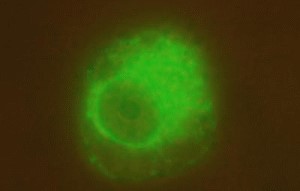
A fluorescent image of the cell
taken four hours into the same experiment. At this time the
quantum dot-siRNA complex is distributed throughout the cellular
fluid. The dark region in the middle of the cell is the nucleus.
Images by The University of Washington |
"This work helps to overcome the longstanding barrier in the siRNA field: How to achieve high silencing efficiency with low toxicity," said co-author Shuming Nie, a professor in the Wallace H. Coulter Department of Biomedical Engineering, jointly affiliated with the Georgia Institute of Technology and Emory University. Other co-authors are Maksym Yezhelyev and Ruth O'Regan at Emory and Lifeng Qi at the UW. Short pieces of RNA, the working copy of DNA, can disable production of a protein by silencing, or deactivating, a stretch of genetic code. Research laboratories regularly use the technique to figure out what a particular gene does. In the body, RNA interference could be used to treat conditions ranging from breast cancer to deteriorating eyesight. The recent experiments used quantum dots, fluorescent balls of semiconductor material just six nanometers across (lining up 9,000 dots end to end would equal the width of a human hair). Quantum dots' unique optical properties cause them to emit light of different colors depending on their size. The dots are being developed for cellular imaging, solar cells and light-emitting diodes. This paper describes one of the first applications of quantum dots to drug delivery. Each quantum dot was surrounded by a proton sponge that carried a positive charge. Without any quantum dots attached, the siRNA's negative charge would prevent it from penetrating a cell's wall. With the quantum-dot chaperone, the more weakly charged siRNA complex crosses the cellular wall, escapes from the endosome (a fatty bubble that surrounds incoming material) and accumulates in the cellular fluid, where it can do its work disrupting protein manufacture. Key to the newly published approach is that researchers can adjust the chemical makeup of the quantum dot's proton-sponge coating, allowing the scientists to precisely control how tightly the dots attach to the siRNA. Quantum dots were dramatically better than existing techniques at stopping gene activity. In experiments, a cell's production of a test protein dropped to 2 percent when siRNA was delivered with quantum dots. By contrast, the test protein was produced at 13 percent to 51 percent of normal levels when the siRNA was delivered with one of three commercial reagents, or reaction-causing substances, now commonly used in laboratories. Central to the finding is that fluorescent quantum dots allow scientists to watch the siRNA's movements. Previous siRNA trackers gave off light for less than a minute, while quantum dots, developed for imaging, emit light for hours at a time. In the experiments the authors were able to watch the process for many hours to track the gene-silencer's path. The new approach is also five to 10 times less toxic to the cell than existing chemicals, meaning the quantum dot chaperones are less likely to harm cells. The ideal delivery vehicle would have no effect; the only biological change would be siRNA blocking cells' production of an unwanted protein. The exact reason that the quantum dots were more effective than previous techniques is, however, still a mystery. "We believe the improvement is caused by the endosome escape, and the ability of the quantum dots to separate from the siRNA," Gao said. Quantum dots are not yet approved for use in humans. The authors are now transferring their techniques to particles of iron oxide, several types of which have been approved by the Food and Drug Administration for use in humans. They are also working to target cancer cells by attaching to specific markers on the cells' surface. "Looking forward, this work will have important implications in in-vivo siRNA therapeutics, which will require the use of nontoxic iron oxide and biodegradable polymeric carriers rather than quantum dots," Nie said.
|